Celebrating 10 Years of Suzaku
The Origin and Heating Mechanism of Supernova Remnants
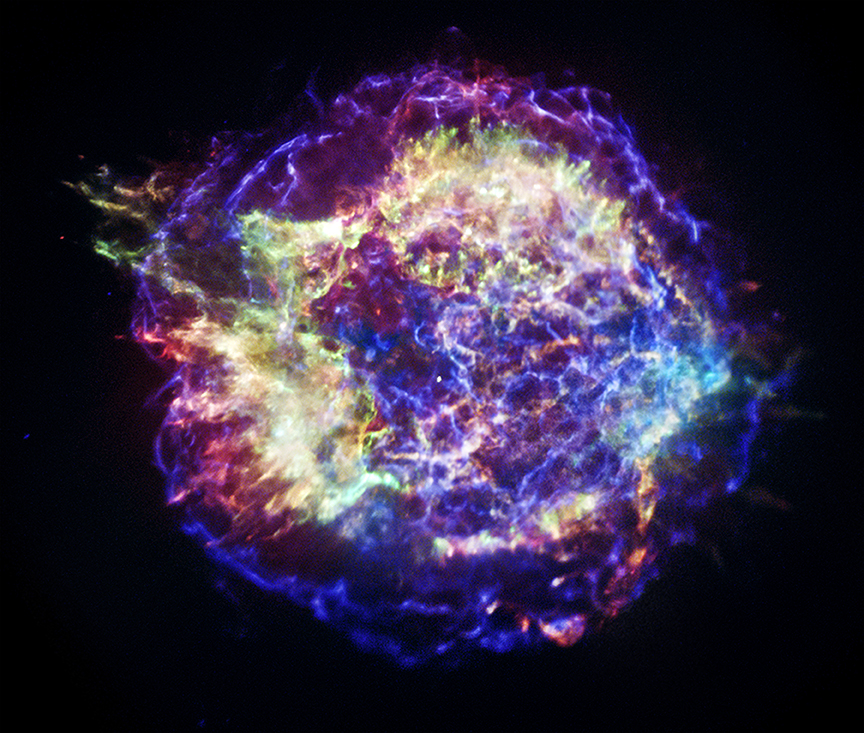
Million-second observation of supernova remnant Cassiopeia A taken by the Chandra X-ray Observatory in 2004. (Credit: NASA/CXC/GSFC/U. Hwang et al.)
All stars fuse lighter elements into heavier ones, producing energy to combat the force of gravity. Stars more massive than about 8 times the mass of the Sun will go through many stages of nuclear fusion in the core from hydrogen to helium, from helium to carbon, and on and on until it accumulates a core of iron. This is where energy-generating fusion reaction stops. Once the iron core grows beyond a certain mass (about 1.4 times the mass of the Sun, called the Chandrasekhar limit), it cannot support its own weight and collapses. The envelope of the star falls onto the collapsing core, and rebounds: this is a core-collapse supernova.
In a star like the Sun, on the other hand, nuclear fusion stops far short of iron. A carbon-oxygen core is created, which does not achieve a high enough temperature and pressure to proceed to the next stage of fusion. Such a star sheds the outer layers far more gently, leaving behind a white dwarf star. However, if a white dwarf is in a binary system, it can explode as a different type (thermonuclear, or Type Ia) supernova. Over the last 20 years or so, cosmologists have used a comparison of the apparent brightness and the cosmological redshift of Type Ia supernovae to infer that the expansion of the universe is accelerating.
But how exactly do white dwarfs become Type Ia supernovae? There are two broad categories of models. In one, two white dwarf stars in a tight binary are brought together, as their orbit decays due to gravitational radiation. In the other, the binary consists of a white dwarf and a relatively normal star, and the white dwarf accretes matter from its companion; as its mass grows to near the Chandrasekhar limit, its core temperature and pressure increase till it explodes. Is one scenario right and the other wrong, or are both scenarios possible? If the latter, could that potentially skew the cosmological results?
In both types, the supernova leaves behind an expanding cloud of gas, which collides with the interstellar medium and gets heated to X-ray emitting temperatures over the subsequent centuries and millennia. X-ray observations of these supernova remnants (SNRs) can help us understand the details of the explosions and subsequent collisions, as well as about the progenitors the stars that became the supernovae. In particular, high temperature leads to ionization stripping of electrons from atoms as they collide with each other and with electrons. Each ionization stage of each element has a characteristic energy X-rays are frequently emitted at that energy, leading to "emission lines". The Suzaku XIS can detect weak lines better than Chandra or XMM-Newton, and it can also determine the precise energy of lines better than other X-ray telescopes.
This improved ability of Suzaku has led to several major discoveries, including these three top "hits".
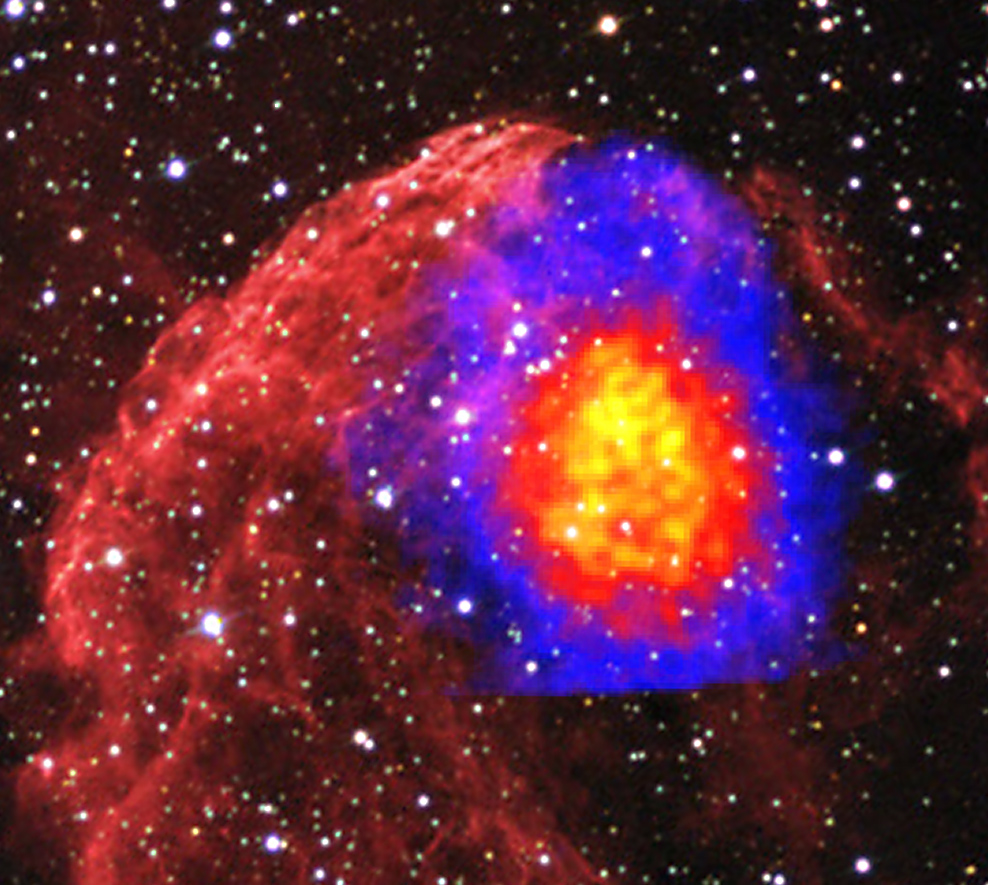
Close-up image of the X-ray emission in the supernova remnant known as the Jellyfish Nebula. (Credit: JAXA/NASA/Suzaku, Tom Bash and John Fox/Adam Block/NOAO/AURA/NSF)
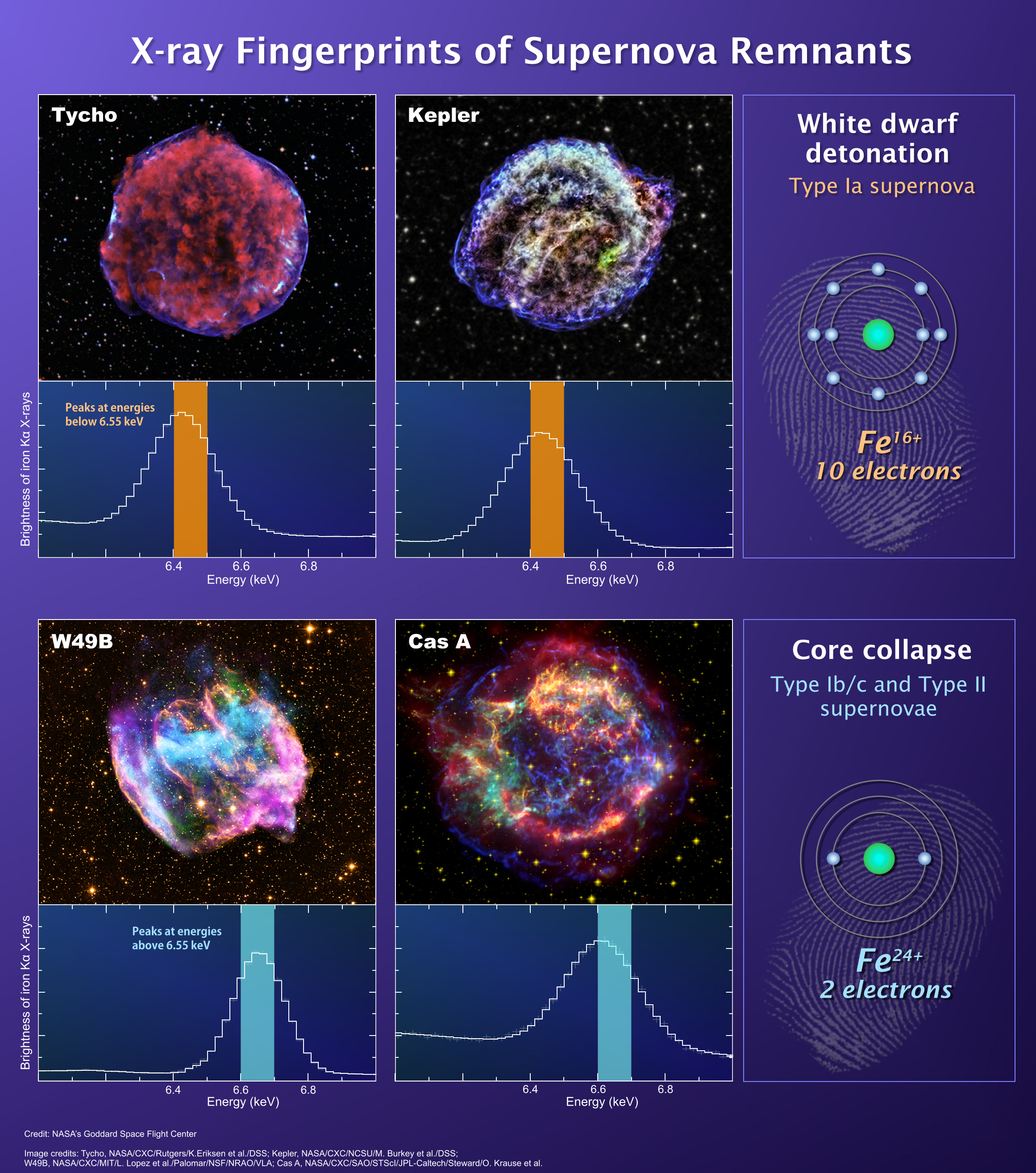
Suzaku observations of 23 supernova remnants, including those shown here, reveal a distinction between those from massive stars and those from white dwarfs. (Credits: NASA's Goddard Space Flight Center)
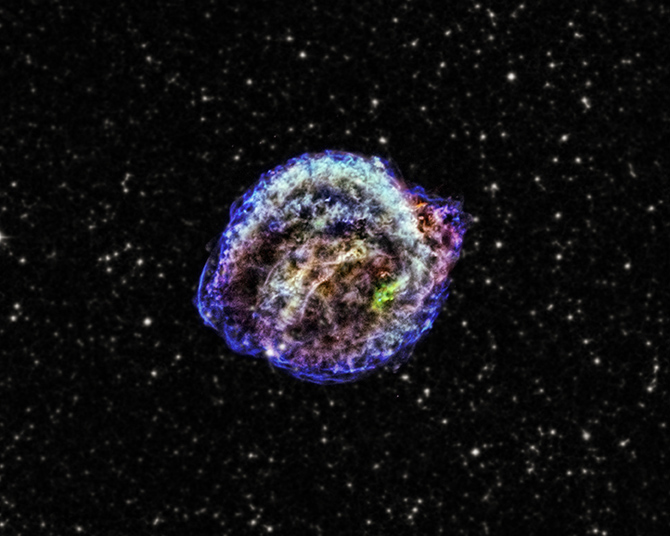
Composite of image of Kepler's Supernova in low (red), intermediate (green) and high-energy (blue) X-rays. The background is an optical star field taken from the Digitized Sky Survey. (Credit: X-ray: NASA/CXC/NCSU/M.Burkey et al.; optical: DSS)
Publication Date: July 2015