Brief History of Timing Analysis
Brief History of Timing Analysis
Timing celestial events has been very important in the history of human culture. Let us look at a few examples.
The First Calendars
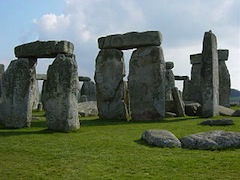
Stonehenge
Early farming societies were at the mercy of the seasons. In order for a group of people to sustain a successful farming culture, they needed to come up with accurate ways of predicting when spring would occur (to know when to plant) and when the first frost could be expected (to know when to harvest). Early civilizations recognized repeating patterns that the Sun and stars made in the sky. They soon realized that the cycles they saw in the sky often coincided with the seasons.
Patterns that stars make, called constellations, rise slightly earlier each night for part of the year; they come back to the same place in the sky after a full year has passed. The highest position of the Sun in the sky also goes through a repeating pattern, reaching its highest point on the first day of summer and its lowest point on the first day of winter. Early civilizations built elaborate constructs, such as Stonehenge in England, to precisely determine when the Sun's position had reached these points in its path on the sky. By understanding and predicting the seasons in this way, they were able to succeed at farming. This is an early example of the importance of timing in astronomy.
Timing Eclipses
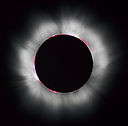
Total solar eclipse
Credit: Image courtesy of Luc Viatour
Early astronomers came up with ingenious ways of finding out information about the Earth, Moon, and Sun from careful observations using accurate eclipse times. During a lunar eclipse, the Moon passes into the Earth's shadow for a while, blocking reflected sunlight which normally causes the Moon to shine brightly. Early astronomers realized that they could find out the relative sizes of the Earth and the Moon by timing how long the Moon stayed in the Earth's shadow during a lunar eclipse. Modern astronomers use a similar method today to estimate the diameters of stars in binary (double) star systems.
Serendipity
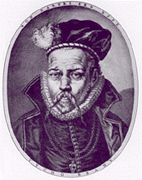
Astronomer Tycho Brahe.
The Danish astronomer Tycho Brahe was very careful about making accurate position and timing measurements of objects in the sky, particularly the planets. He designed an elaborate observatory for this purpose, and during many years of observations he collected a great wealth of position and timing data on the planets. Years after his death, other astronomers (such as Kepler and Newton) used these precise data to develop empirical laws describing the motions of the planets, and theories of the physics of motion. Had Brahe not collected such an impressive array of information, who knows how long it would have taken for these basic laws of physics to be understood?
Measuring the Speed of Light
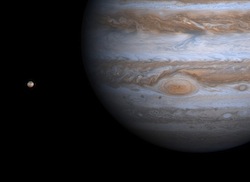
Jupiter and one of its moons, Io.
Credit: NASA/JPL/University of Arizona
Timing the motions of the satellites of Jupiter led astronomers to one of the first ways to measure the speed of light. Astronomers noticed that when Jupiter and Earth were close to each other on their orbits about the Sun, Jupiter's satellites would pass in front of the planet a few minutes ahead of the predicted time. When the two planets were far separated on their orbits, the transits occurred slightly later than expected. The difference arose from the fact that the information travels at the speed of light, and the distance from Jupiter to Earth is not constant, but varies by a few light minutes depending on where in their orbits the two planets are. Realizing this, astronomers calculated a reasonably accurate value for the speed of light by timing the transits of Jupiter's moons at different points along its orbit.
Sunspots
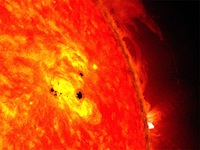
Two sunspots observed by NASA's Solar Dynamics Observatory
Credit: NASA/SDO/AIA/HMI/Goddard Space Flight Center
When astronomers look at the Sun with special filters, they see that it is not just a uniformly bright ball of hot gas but is frequently covered with dark spots of various sizes and shapes, called sunspots. The number of sunspots, and their location on the Sun's surface, changes in a regular way, repeating itself roughly every 11 years. Early Chinese astronomers took note of the sunspot number as early as 28 B.C. Astronomers have learned much information about the magnetic field of the Sun and its interaction with Earth through accurate observations of the sunspot number and how this correlates with magnetic disturbances on Earth.
Astronomers use the same basic principles of timing regular changes in various characteristics of cosmic objects to gain information about objects outside the Solar System, as well.
X-ray Binaries
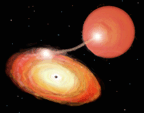
An artist's conception of an X-ray pulsar
in a binary star system.
Credit: M. Masetti, NASA/GSFC
An X-ray binary system is a pair of stars in orbit about one another, where one of the stars is a compact object (a white dwarf, neutron star, or black hole) and the other is a normal star. Material from the normal star accretes onto the surface of the compact companion, heats, and emits X-rays that we detect on Earth. X-ray binaries typically exhibit a wide range of variability, particular in the X-ray region of the electromagnetic spectrum.
One type of variation is periodic. When two stars orbit each other, there are three "clocks" in the system, which each give rise to a regular period. These clocks, which are also present in the Sun-Earth system, are 1) the ROTATION of the compact object, 2) the ORBITAL MOTION of the stars about their common center of mass and 3) the PRECESSION of an accretion disk, a thin disk of material from the normal star orbiting about the compact companion. This disk is often tilted and twisted, and can shadow the X-ray source periodically.
Pulsars, Doppler Shifts, and Neutron Star Masses
When a pulsar is in a binary system, its motion about the center of mass of the system can be measured from the Doppler shift of its pulse period. During the part of the orbit when the pulsar is moving toward observers on the Earth, the period appears to be shorter, and when it is moving away the period appears longer. In some X-ray binary systems, the motion of the normal star can also be measured by the Doppler shift in the spectral lines in the optical. Using this information from both stars, we can solve for the masses of both the normal star and the compact object. This has important consequences for theories of stellar evolution, which predict a relatively narrow range of masses that neutron stars are allowed to have.
Neutron Star Spin-down and Age
Many pulsars have now been observed for decades. It turns out that isolated neutron stars are not spinning at a constant rate but are instead slowing down very gradually. This slowing is called the spin-down rate, and it is a measure of the amount of energy the pulsar is losing as a function of time, and hence the age of the pulsar. Different models of the magnetic structure of pulsars predict different spin-down rates. Through long-term monitoring of the spin-down rates of isolated pulsars, astronomers can determine which of the models best predicts the observed behavior.
Aperiodic Variations
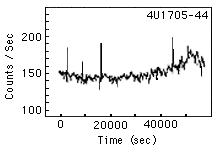
A light curve that shows aperiodic variations
Variability that appears more random on a variety of timescales is even more common, and may even be universal, in X-ray binary systems. Changes on the order of months might represent changes in the accretion rate or evolution of the accretion disk. Flares of a few seconds may indicate differences in the accretion flow, or thermonuclear flashes on the surface of the neutron star. Some sources show surprisingly erratic variations, sometimes with flares as short as milliseconds. The underlying physics governing this process is still not well understood. On the right is a light curve showing aperiodic variation in X-ray intensity.
QPOs and X-ray Binaries
Some X-ray binary systems display a complicated variation which is termed "quasi-periodic oscillations" or QPOs for short. When astronomers study these systems, they find variations over a close range of periods, usually centered around one value. The mechanism giving rise to this almost, but not quite, periodic behavior is still a mystery. Many researchers believe they are caused by the interaction of the accretion disk around a compact object and the magnetic field near the object. Material at different distances from the compact object would explain why an entire range of periods is observed. Continued monitoring of QPOs in various types of X-ray binary systems will allow astronomers to refine the models which give rise to this behavior.
Updated: August 2013
