Cosmic Rays
Cosmic Rays
Cosmic rays provide one of our few direct samples of matter from outside the solar system. They are high energy particles that move through space at nearly the speed of light. Most cosmic rays are atomic nuclei stripped of their atoms with protons (hydrogen nuclei) being the most abundant type but nuclei of elements as heavy as lead have been measured. Within cosmic-rays however we also find other sub-atomic particles like neutrons electrons and neutrinos.
Since cosmic rays are charged positively charged protons or nuclei, or negatively charged electrons their paths through space can be deflected by magnetic fields (except for the highest energy cosmic rays). On their journey to Earth, the magnetic fields of the galaxy, the solar system, and the Earth scramble their flight paths so much that we can no longer know exactly where they came from. That means we have to determine where cosmic rays come from by indirect means.
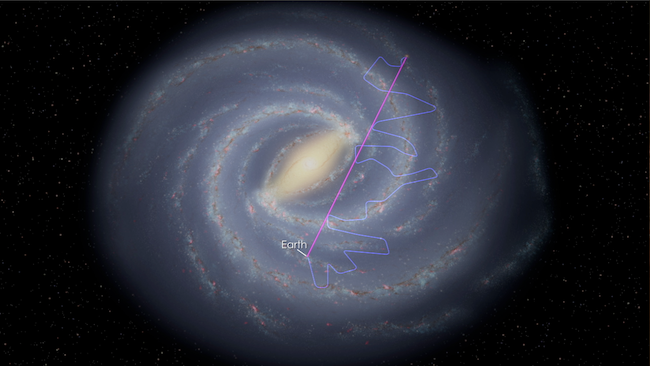
Because cosmic rays carry electric charge, their direction changes as they travel through magnetic fields. By the time the particles reach us, their paths are completely scrambled, as shown by the blue path. We can't trace them back to their sources. Light travels to us straight from their sources, as shown by the purple path. (Credit: NASA's Goddard Space Flight Center)
One way we learn about cosmic rays is by studying their composition. What are they made of? What fraction are electrons? protons (often referred to as hydrogen nuclei)? helium nuclei? other nuclei from elements on the periodic table? Measuring the quantity of each different element is relatively easy, since the different charges of each nucleus give very different signatures. Harder to measure, but a better fingerprint, is the isotopic composition (nuclei of the same element but with different numbers of neutrons). To tell the isotopes apart involves, in effect, weighing each atomic nucleus that enters the cosmic ray detector.
All of the natural elements in the periodic table are present in cosmic rays. This includes elements lighter than iron, which are produced in stars, and heavier elements that are produced in violent conditions, such as a supernova at the end of a massive star's life.
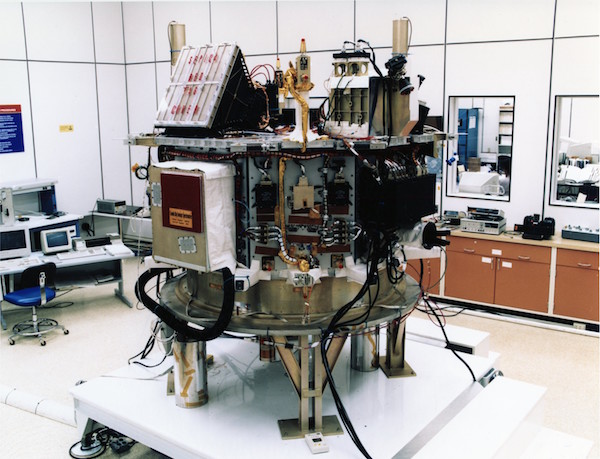
The Cosmic Ray Isotope Spectrometer (CRIS - the box on the left of the spacecraft, with a yellow label) on the Advanced Composition Explorer (ACE) spacecraft provides measurements of the isotopes of galactic cosmic ray nuclei ranging from helium to zinc. ACE launched in August 1997. (Credit: NASA/Johns Hopkins University Applied Physics Laboratory)
Detailed differences in their abundances can tell us about cosmic ray sources and their trip through the galaxy. About 90% of the cosmic ray nuclei are hydrogen (protons), about 9% are helium (alpha particles), and all of the rest of the elements make up only 1%. Even in this one percent there are very rare elements and isotopes. Elements heavier than iron are significantly more rare in the cosmic-ray flux but measuring them yields critical information to understand the source material and acceleration of cosmic rays
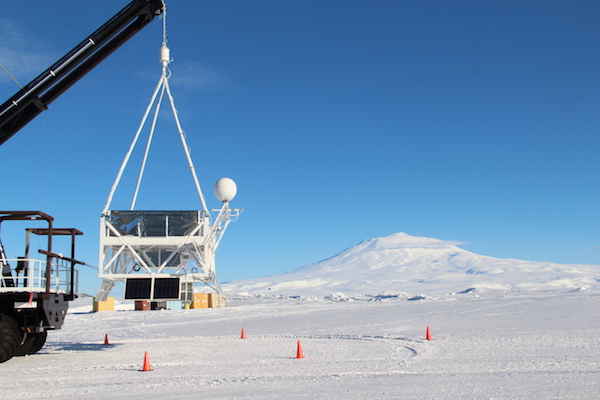
SuperTIGER hanging from the launch vehicle just before its December 2012 launch with Mount Erebus in the background. SuperTIGER is a cosmic-ray balloon instrument measuring cosmic-rays heavier than iron to explore the source of cosmic rays and their acceleration sites. SuperTIGER's first flight lasted for 55 days, a record in duration for an Antarctic long duration scientific balloon payload. (Credit: Ryan Murphy/Washington University)
Even if we can't trace cosmic rays directly to a source, they can still tell us about cosmic objects. Most galactic cosmic rays are probably accelerated in the blast waves of supernova remnants. The remnants of the explosions expanding clouds of gas and magnetic field can last for thousands of years, and this is where cosmic rays are accelerated. Bouncing back and forth in the magnetic field of the remnant randomly lets some of the particles gain energy, and become cosmic rays. Eventually they build up enough speed that the remnant can no longer contain them, and they escape into the galaxy.
Cosmic rays accelerated in supernova remnants can only reach a certain maximum energy, which depends on the size of the acceleration region and the magnetic field strength. However, cosmic rays have been observed at much higher energies than supernova remnants can generate, and where these ultra-high-energies come from is an open big question in astronomy. Perhaps they come from outside the galaxy, from active galactic nuclei, quasars or gamma ray bursts. Or perhaps they're the signature of some exotic new physics: superstrings, exotic dark matter, strongly-interacting neutrinos, or topological defects in the very structure of the universe. Questions like these tie cosmic-ray astrophysics to basic particle physics and the fundamental nature of the universe.
Confined by a magnetic field in supernova remnants, high-energy particles move around randomly. Sometimes they cross the shock wave. With each round trip, they gain about 1 percent of their original energy. After dozens to hundreds of crossings, the particle is moving near the speed of light and is finally able to escape. (Credit: NASA's Goddard Space Flight Center)
Text updated: July 2017
